Photoreceptor cells in our eyes can adjust to both weak and strong light levels, but we still don’t know exactly how they do it. Emeritus Professor Fumio Hayashi of Kobe University and his colleagues revealed that the photoreceptor protein rhodopsin forms transient clusters within the disc membranes in retina. These clusters are concentrated in the center of disc membranes, and act as platforms in the process of light to chemical signal conversion. The findings were published as a highlighted paper in Communications Biology on June 14.
The research team for this study include Associate Professor Kenichi Morigaki (Biosignal Research Center, Kobe University), Emeritus Professor Shohei Maekawa (Graduate School of Science, Kobe University), Associate Professor Keiji Seno (Faculty of Medicine, Hamamatsu University School of Medicine) and Researcher Natsumi Saito (School of Medicine, Jichi Medical University).
Within the rod-shaped photoreceptor cells in our retinas there are roughly 1,000 layers of disc-shaped membranes, a few micrometers in diameter. These are lipid bilayer membranes embedded with high concentrations of the photoreceptor protein rhodopsin. Rhodopsin is a prototypical member of G protein-coupled-receptor (GPCR) that is responsible for collecting various information about our external environment.
In this study, researchers used the latest technology and analysis methods to investigate the single molecule dynamics of rhodopsin and G protein transducin as well as lipid molecules within disc membranes.
Single-molecule tracking of rhodopsin
Rhodopsin is sensitive to light, so instead of using the standard visible light fluorescent dye the team used near-infrared dye for the single-molecule tracking. They confirmed that rhodopsin moves quite freely within disc membranes, and predicted that if rhodopsin is forming temporary clusters, the molecule would move from moment to moment at different speeds. They collected 500 individual “tracks” created by the rhodopsin movements (Figure 1 left), obtained a three-state model by using Bayes inference machine learning technology (Figure 1 center) and found that rhodopsin transitions between three diffusive states (Figure 1 right).
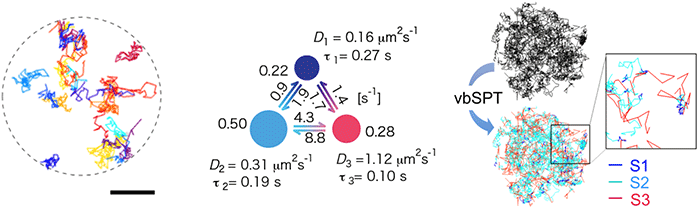
Left: tracks of rhodopsin diffusion in the disc membrane (each molecule is a different color). Center: dynamic equilibrium model of the three patterns obtained using machine learning based on Bayes theory.
Right: the three patterns seen in rhodopsin tracks in the disc membrane (red: fast, light blue: medium, dark blue: slow).
Clusters that form and disappear
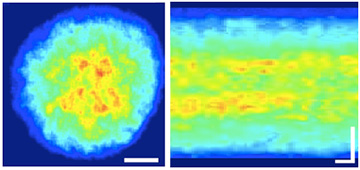
Left: Rhodopsin clusters in the center of the disc membrane (still image). Scale bar: 2 micrometers.
Right: Time lapse of rhodopsin density. Scale bar: horizontal 0.1 seconds, vertical 2 micrometers.
When they increased the fluorescent marking of rhodopsin, the team was able to observe the repeated generation and extinction of rhodopsin clusters (Figure 2). Properties such as the diffusion speed and lifespan of the clusters matched with the slowest diffusion observed in single-molecule tracking. The team also discovered that rhodopsin distribution within disc membranes is not uniform as previously thought: they are spread thinly around the edges and concentrated in the center, where they easily form clusters.
Inequality between the center and the periphery
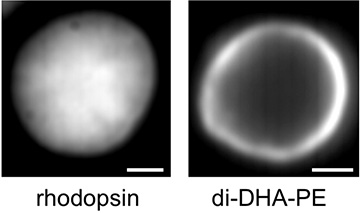
Left: Distribution of rhodopsin biased towards the center of the disc membrane (equilibrium image). Right: Distribution of raftophobic lipid di-DHA-PE biased towards the edges of the disc membrane (equilibrium image).
The raft-forming nature of rhodopsin molecules when they draw close to each other suggests that rhodopsin clusters are similar to rafts. The distribution of rhodopsin in disc membranes is weighted towards the center (Figure 3 left), indicating that raftophilic proteins and lipid molecules gather in the center of the disc membrane, whereas raftophobic molecules tend to gather at the edges. The team verified this by showing that the typical raftophobic phospholipid di-DHA-PE is localized at the membrane edges (Figure 3 right).
Summary
The study demonstrates that (1) rhodopsin makes raftophilic clusters, providing a transient scaffold for the activation of G-protein signal transducing, and (2) rhodopsin clusters are rejected from the periphery of membranes, and collect in the center. These discoveries suggest that both transient non-uniformity and structural non-uniformity regulated by the surrounding framework play unexpectedly important roles, not only in this case but also in various other cell membranes.
This provides a vital piece of very basic knowledge. Developments in basic research are essential for understanding the complex morphogenesis and maintenance of photoreceptors, as well as pathological conditions such as retina pigment abnormalities. In this study the team focused on molecules related to the activation process of light signal conversion, but the deactivation process is also an unknown domain. We will continue to pursue further developments in research on both the activation and deactivation processes.
Journal Information
- Title
- “Raftophilic rhodopsin-clusters offer stochastic platforms for G protein signalling in retinal discs”
- DOI
- 10.1038/s42003-019-0459-6
- Authors
- Fumio Hayashi1, Natsumi Saito1†, Yasushi Tanimoto2, Keisuke Okada2, Kenichi Morigaki2,3, Keiji Seno4, Shohei Maekawa1
1 Graduate School of Science, Kobe University
2 Graduate School of Agricultural Science, Kobe University
3 Biosignal Research Center, Kobe University
4 Faculty of Medicine, Hamamatsu University School of Medicine
† Current employment: Researcher at the School of Medicine, Jichi Medical University - Journal
- Communications Biology