A multi-institutional research collaboration has revealed that the microtubule network (*1), which plays a vital role in cell construction and polarization (*2), utilizes the phase separation (*3) properties of CAMSAP2 proteins in its formation. To illuminate this process, they used cryo-electron microscopy (*4) and total internal reflection fluorescence microscopy (*5), among other methods.
Professor NITTA Ryo and Assistant Professor IMASAKI Tsuyoshi et al.’s research group at Kobe University’s Graduate School of Medicine collaborated with Visiting Senior Scientist TAKEICHI Masatoshi (RIKEN emeritus researcher) of the RIKEN Center for Biosystems Dynamics Research, Researcher SHIGEMATSU Hideki of the RIKEN Spring-8 Life Science Research Infrastructure Group (at the time of this study, he was a researcher at the Materials and Structural Analysis laboratory of the Japan Synchrotron Radiation Research Institute (JASRI)) and Associate Professor NIWA Shinsuke (Frontier Research Institute for Interdisciplinary Sciences, Tohoku University).
These results explain the fundamental mechanism by which some types of cell obtain their shape (such as myocardial (heart muscle) cells, nerve cells, epithelial cells, and fibroblasts). This discovery will have a wide ranging impact across a variety of fields, for example in medicine this new understanding of microtubule formation could inform research into diseases that can be caused by abnormalities in non-centrosomal microtubule networks (such as cancer and neurodegenerative diseases).
These research results were published online in the international academic journal eLife on June 28, 2022.
Main points
- Using its phase separation property, CAMSAP2 takes in and co-condensates with tubulin (a protein that makes microtubules) to nucleate countless microtubules in a short amount of time. This becomes a microtubule nucleation center with the microtubules extending radially to form Cam2-aster (*6).
- The formation of a microtubule network (which begins with CAMSAP2) is important for understanding how cell polarization and cell maintenance work when they are driven by microtubule networks that do not depend on centrosomes (for example, in cardiomyocytes, nerve cells, epithelial cells, and fibroblasts).
Research Background
The microtubule network is a highly organized cytoskeleton located inside a cell. It plays a vital role in maintaining cell polarization and structure. For many years, it was thought that microtubule network formation is controlled by y-tubulin ring complex (Y-TuRC), a unique microtubule-organizing center (MTOC). However more recent research has reported that network formation in highly polarized cells (i.e. those with very specialized jobs like cardiomyocytes, nerve cells, epithelial cells and fibroblasts) occurs without Y-TuRC; in other words they have non-centrosomal microtubule networks. Consequently, how such microtubule networks form has received much attention.
There are three families of CAMSAPs (calmodulin-regulated spectrin-associated proteins) found in mammals. Like y-TuRC, these proteins bind the beginning end of microtubule elongation. Studies have reported that CAMSAP2 is important for the formation of asymmetry in zebrafish, and that deficient or dysfunctional CAMSAP3 disturbs the organization of microtubules, causing abnormal epithelial cell morphology and function. Since CAMSAP binds to a microtubule’s elongated end and is also involved in cell polarization, the current study focused on CAMSAP as a potential y-TuRC-independent MTOC regulator.
Research Methodology
Microtubules are supramolecular complexes that function as a cytoskeleton and also transport cargo inside the cell. These hollow tube-like structures are composed of tubulin molecules. If a concentration of over 20 µM of tubulin is incubated at 37°C, tubulin molecules naturally polymerize into microtubules (hereafter, referred to as polymerization). On the other hand, the concentration of tubulin in a cell is only a few µM. However, microtubules can be formed with the aid of proteins that organize a microtubule nucleation center. It is known that the predominantly centrosomal protein complex y-TuRC can organize microtubule nucleation centers, however it was not understood how microtubules form without centrosomes (hereafter referred to as non-centrosomal).
This research group focused on the protein CAMSAP2, which, like y-TuRC, binds to microtubules to stabilize their minus ends. They conducted experiments where they mixed CAMSAP2 and tubulin in test tubes. When only tubulin was present, microtubule formation couldn’t occur if the concentration was under 20µM, however they discovered that when CAMSAP2 was added, microtubule formation occurred at 1 -2 µM, which is a lower concentration than that found inside a cell. The research group subsequently observed the dynamics of CAMSAP2 under a fluorescence microscope and discovered that CAMSAP2 condensates through phase separation to form an oil droplet. This oil droplet selectively takes in tubulin, which increases the local concentration of tubulin. Temporally observing the mixture of CAMSAP2 and tubulin at 37°C via total internal reflection fluorescence microscopy revealed that microtubules began to radially extend from the oil droplet that CAMSAP2 took tubulin into, which led to the subsequent formation of a microtubule network-like structure. The research group named this structure Cam2-aster because its appearance strongly resembles the aster structure of a centrosome. To elucidate further, the researchers observed this process in more detail using their laboratory’s electron microscope (EM) and the high-end cryo-EM at the SPring-8 facility and Osaka University’s Research Center for Ultra-High Voltage Electron Microscopy. They succeeded in observing the intermediate structures. After CAMSAP2 co-condensates with tubulin, the Cam2-aster structure forms in a very short period of time. During this process, the tubulin molecules are longitudinally connected to form tubulin-rings and these tubulin-ring structures are laterally connected to form sheet-like structures, which then became microtubules. Thus, the researchers propose the following mechanism: CAMSAP2 uses phase separation to create a MTOC, within which the formation of intermediate structures and microtubules is promoted, and then Cam2-aster mediates the formation of a microtubule network (see figure).
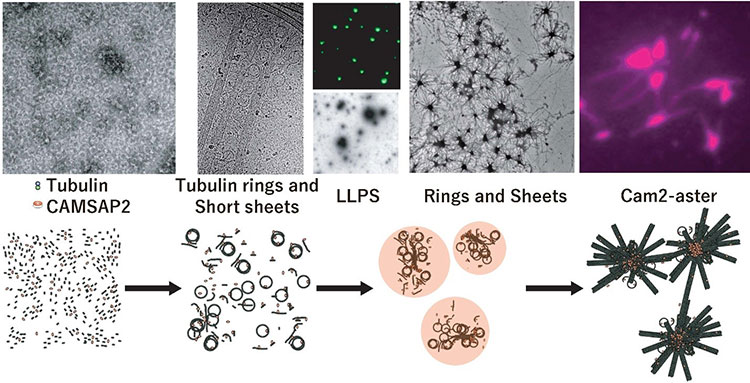
Top: CAMSAP2-mediated polymerization of reconstituted structures in test tubes as observed via electron microscopy, fluorescence microscopy and total internal reflection fluorescence microscopy, respectively.
Below: Model of CAMSAP2-mediated microtubule polymerization/network formation. The following phenomena were observed: tubulin connecting vertically, curling to form ring shapes (tubulin rings), and tubulin protofilaments being integrated side-by-side to form sheets.
Key: Green: CAMSAP2, Magenta: Tubulin. LLPS: Liquid-Liquid Phase Separation.
Further Research
The discovery that CAMSAP induces non-centrosomal microtubule networks is vital to our understanding of the fundamental mechanism behind cell polarization (in cardiomyocytes, nerve cells, epithelial cells, and fibroblasts etc.), in which microtubule networks play an important role. For example, non-centrosomal microtubule networks play a key role in the stable formation of cardiomyocyte’s characteristic cylindrical shape and give it resistance to contraction. Therefore, it is expected that the importance of this study’s findings will influence a wide range of fields including neurology, cell biology, developmental biology, structural biology and cardiology. In addition, abnormalities in non-centrosomal microtubule networks are known to cause heart failure, neurodegenerative disorders and cancer among other diseases. Consequently, this newly discovered mechanism behind microtubule formation could be an important step towards illuminating disease pathologies and developing new treatments. Also, the researchers succeeded in observing numerous intermediate states in the microtubule formation process, which greatly benefits our molecular understanding of how microtubules extend from tubulin.
Glossary
1. Microtubule network
A network of microtubules that form the ‘skeleton’ of a cell (cytoskeleton), enabling it to keep its shape and also functioning as a pathway for transporting substances throughout the cell.
2. Cell polarization
Molecules are unevenly distributed throughout a cell- this phenomenon is called polarity and enables cells to differentiate into types. The molecule actin in the cytoskeleton and the microtubules play important roles in cell polarization.
3. Phase separation
Phase separation is a phenomenon where two liquids do not mix together but instead separate into two phases. Research in recent years has shown that phase separation occurs when some kinds of protein are submerged in water- they separate from the water similar to how oil does. When phase separation of proteins occurs in an aqueous liquid, the proteins assemble in what looks like a drop of oil to form a chemical reaction site, exhibiting their specific function.
4. Cryo-electron microscopy
This is a type of electron microscopy technique, which was developed so that biological samples such as nucleic acid and proteins could be observed. The three researchers who developed the technology for the cryo-electron microscope won the 2017 Nobel Prize in Chemistry. The liquid containing the biological sample is thinly spread out and rapidly frozen in liquid ethane (-183°C~ -160°C). Thus the sample is trapped inside a thin layer of ice and is viewed by an electron microscope at the temperature of liquid nitrogen (-196°C). Compared to methods where a sample is fixed and stained, less damage is done to the sample by the electron beam due to the low temperature at which it is viewed under the microscope. Other advantages of cryo-electron microscopy include the fact that it is possible to observe a sample in a fluid state.
5. Total internal reflection fluorescence microscopy
A fluorescence microscope technique that uses evanescent light produced from a tunneling effect as its excitation source. It has a higher resolution than an optical microscope and allows microtubules and the proteins that bind to microtubules to be viewed on a unimolecular level.
6. Cam2-aster
CAMSAP2 co-condensates with tubulin to induce microtubule elongation, which results in the microtubules radiating outwards to form an aster-like structure that resembles a centrosome. This research group named this aster-like structure Cam2-aster.
Acknowledgements
This research received funding from the following:
- Japan Agency for Medical Research and Development (JP21gm0810013 and JP21gm1610003)
- Japan Science and Technology Agency (JPMJPR14L2)
- Moonshot Research and Development Program (JPMJMS2024)
- Takeda Science Foundation
- Mochida Memorial Foundation for Medical and Pharmaceutical Research
- Uehara Memorial Foundation
- Bristol-Myers Squibb
- Hyogo Science and Technology Association
- Japan Society for the Promotion of Science (KAKENHI) (Grant numbers: 19K07246, 25221104, 19H03396, 21H05254)
- FOREST Program (JPMJFR214K)
Journal Information
Title
“CAMSAP2 organizes a γ-tubulin-independent microtubule nucleation centre”
DOI
10.7554/eLife.77365
Authors
Tsuyoshi Imasaki1-3†, Satoshi Kikkawa1†, Shinsuke Niwa4*, Yumiko Saijo-Hamano1, Hideki Shigematsu5, Kazuhiro Aoyama6,7, Kaoru Mitsuoka7, Takahiro Shimizu1, Mari Aoki3, Ayako Sakamoto3, Yuri Tomabechi3, Naoki Sakai5, Mikako Shirouzu3, Shinya Taguchi1, Yosuke Yamagishi1, Tomiyoshi Setsu1, Yoshiaki Sakihama1, Eriko Nitta1, Masatoshi Takeichi8, Ryo Nitta1,3*
1 Division of Structural Medicine and Anatomy, Department of Physiology and Cell Biology, Kobe University Graduate School of Medicine
2 JST, PRESTO
3 RIKEN Center for Biosystems Dynamics Research
4 Frontier Research Institute for Interdisciplinary Sciences, Tohoku University
5 RIKEN SPring-8 Center, Hyogo, 679-5148, Japan
6 Materials and Structural Analysis (ex FEI), Thermo Fisher Scientific
7 Research Center for Ultra-High-Voltage Electron Microscopy, Osaka University
8 RIKEN Center for Biosystems Dynamics Research
† These authors contributed equally to this work
* corresponding authorJournal
eLife